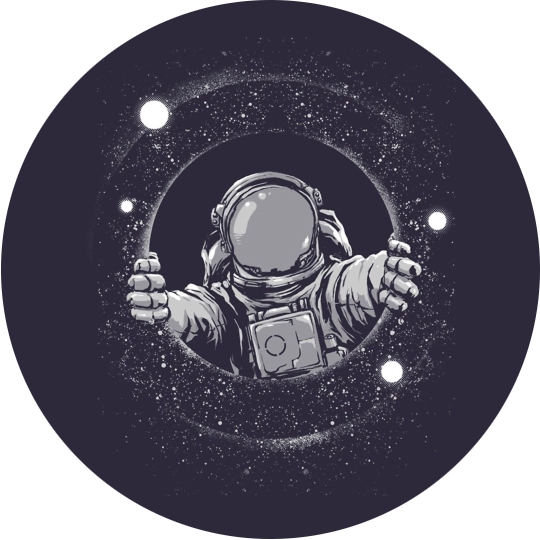
Publication date: May 09, 2024
This article will concentrate on the concept and on the potential the sub0layer could have on Web3. It will not focus on the technical implementation. To understand what the sub0layer is meant to be, it is necessary to examine the general segmentation of the blockchain infrastructure. In the blockchain, a fundamental distinction is made among several layers: Layer 0 (L0), Layer 1 (L1), Layer 2 (L2), and Layer 3 (L3), each performing different functions within the blockchain architecture.
Layer 0 forms the foundational network and communication infrastructure that enables various blockchain protocols to interact with each other and facilitates interoperability among different blockchains. Layer 1 can function either as a standalone blockchain, providing both the network infrastructure and the platform for decentralized applications, or as a blockchain built atop Layer 0, benefiting from the security and interoperability of a higher-level infrastructure such as Polkadot. Layer 2 technologies are developed on top of the existing L1 blockchain and aim to enhance their scalability and efficiency through off-chain transaction processing, while Layer 3, the application layer, is based on L2 technologies (or directly on L1 if no L2 solutions are utilized) and focuses on providing specific features and services that directly address end-users and optimize the user experience within the blockchain ecosystem. Layers 0 and 1 are the fundamental infrastructure layers within the blockchain ecosystem. Layers 2 and 3, on the other hand, are extensions that build on the Layer 1 infrastructure and address specific problems such as scalability and application development without altering the basic blockchain itself. The segmentation and classification of the various blockchain "layers" are complex and constantly evolving. The terminology and associated concepts have evolved with the advancement of blockchain technology and the introduction of new platforms and solutions. This can lead to confusion, especially when attempting to understand and compare the different architectures and functions of these layers.
To simplify the complexity of the segmentation, we take an example where Polkadot (L0), Ethereum (standalone L1), Astar (L1 on L0), and Polygon (L2) represent different layers and approaches within the blockchain ecosystem, each addressing specific challenges and fulfilling different functions. Polkadot (L0) separates the aspects of security and infrastructure from the specific applications and functionalities that operate on individual parachains (L1 on L0). The Layer 0 component (relay chain) of Polkadot takes care of security, interoperability, and validation. This structure allows parachains such as Astar (L1 on L0) to concentrate on specific applications and functionalities without having to concern themselves with basic network functions such as security or consensus mechanisms. Ethereum, conversely, integrates security, interoperability, validation, and the application platform in a standalone Layer 1 blockchain. Within Ethereum, interoperability among DApps is direct, as they all operate on the same blockchain. For external interoperability, however, bridges such as those between Ethereum and other blockchains are required. Scalability is a critical topic for Ethereum, which has been addressed by introducing Polygon (L2) and other rollups that build on Ethereum but process certain operations off-chain to enhance performance and scalability. Meanwhile, Ethereum has also evolved with the introduction of the sharding concept. Sharding aims to increase network capacity by distributing the load across several smaller partitions, known as shards. Each shard can process its own transactions and smart contracts independently, thus reducing the overall load on the network and enhancing the performance rate. However, with the introduction of sharding in Ethereum, one might assume that the need for Layer 2 solutions like Polygon could decrease as the main chain itself becomes more scalable and efficient. Nonetheless, there are several reasons why Layer 2 solutions remain relevant. Layer 2 solutions can address specific needs that may not be directly covered by sharding. For instance, Polygon could still be used for applications that require extremely fast transaction times or need special functions that cannot be efficiently implemented on the main chain. The full implementation and optimization of sharding in Ethereum are expected to take time. During this transition phase and beyond, Layer 2 solutions will likely continue to play an important role in improving performance and user experience. Although sharding can solve some of the problems currently addressed by Layer 2 solutions such as Polygon, it is unlikely to make Polygon and similar technologies obsolete.
It is essential at this point to grasp the similarities and differences between Ethereum shards and Polkadot parachains, as both adhere to the fundamental concept of reducing the load on the main blockchain by distributing tasks and transactions across separate chains. This distribution aids in enhancing the scalability and processing speed of the respective networks. Each shard in Ethereum independently processes a portion of the transactions and data in parallel to the main chain, thus expanding network capacity while leveraging the security provided by the main chain. In contrast, each parachain within Polkadot offers customized functionalities and benefits from the security of the central relay chain. While Ethereum shards primarily focus on improving processing speed by parallelizing data processing, Polkadot’s parachains provide solutions to implement custom governance structures and specific functionalities. In summary, Ethereum shards are designed to increase the overall performance of the blockchain through parallel data processing, whereas Polkadot's parachains offer customizable blockchains tailored to specific needs and capable of functioning independently of each other. Although both systems enhance scalability, they do so through fundamentally different approaches.
In blockchain networks, the processing of transactions and the storage of data is ensured by a distributed network of computers, known as nodes. Each transaction requires computing power to validate the data and ensure the security of the information. Typically, the computing power utilized for this purpose is compensated by transaction fees paid by the network's users. These fees are intended to cover the costs of maintaining the network infrastructure. The efficiency with which transactions are processed and stored largely determines the scalability and performance of the entire network. In most instances, the basic fee is calculated based on the computing power and storage space required to process the transaction. Although the base fee mainly covers computation and storage, transaction fees also contribute to covering other network costs. Network utilization is a crucial factor; if many transactions are executed simultaneously, the demand may exceed the supply of available computing power. In such cases, fees increase as users are willing to pay more to prioritize their transaction processing. The complexity of the transaction also plays a significant role. A simple transaction, such as transferring cryptocurrency from one address to another, requires less computing power than a complex transaction that involves multiple smart contract interactions. The fees increase with the complexity and number of computing operations required. In some blockchains, the fee depends on the size of the transaction in bytes and the available space in a block. Transactions that contain more data require more storage space in a block and thus can result in higher fees. Some networks set minimum fees to protect the network from spam attacks. These minimum fees ensure that it is too costly for attackers to flood the network with non-essential transactions. Polkadot, for instance, has fixed base transaction fees that are independent of network utilization. These fixed fees are typically lower than the variable fees common in networks like Ethereum, which dynamically adjust based on network utilization. Transaction fees in Polkadot are also calculated based on the "weight" of a transaction, which measures the time and resources required to process the transaction. The issue of fairness in transaction fees is complex. Networks strive to create fair conditions through algorithmic methods and market mechanisms, but the perception of fairness can be subjective, especially when users from economically diverse regions have different capabilities to bear high fees.
It is important to note that staking rewards in Proof-of-Stake (PoS) networks are generated differently and have a different basis than transaction fees. In many PoS networks, staking rewards are produced through the creation of new coins or tokens. This creation is often specified in the network's protocol and serves as an incentive for stakers to secure the network. The process is analogous to mining in Proof-of-Work (PoW) systems, where new coins are created as a reward for mining new blocks. In the PoS system, validators who successfully validate a block receive new coins that are issued as part of the block reward. Unlike PoW, where security is achieved through computing power (mining), in PoS, participants secure the network by "staking" their coins. By "locking" their coins in the network, they demonstrate their financial commitment to the network’s health. This reduces the likelihood of malicious activity, as such actions would diminish the value of their staked investments. The creation of new coins as staking rewards leads to controlled inflation. The inflation rate is often set to provide sufficient staking rewards without causing currency value dilution through excessive inflation. This inflation partly compensates stackers for the risk and loss of liquidity they incur by locking their funds in the network. In some blockchain networks, stakers can also receive a portion of the transaction fees as an additional reward. These fees derive from regular network transactions and can vary depending on the network utilization. This combination of newly created coins and transaction fees forms the total reward for the stakers. In summary, transaction fees primarily cover the cost of computation and validation of transactions on the network. These fees serve to compensate the validators or miners for the computing power and resources they expend to process transactions and securely add them to the blockchain. Staking rewards, on the other hand, are part of an incentive system that rewards participants for staking their cryptocurrencies and thereby contributing to network security. This dual system of incentives ensures both the ongoing operation and the integrity of the network.
The sub0layer is conceived as a foundational computing and storage layer, aimed at fostering a more regulated, decentralized, and independent Web3 ecosystem. Unlike conventional cloud solutions, this layer is intended to function as a decentralized computing data center. It is set to be implemented and managed by the Logos network. In this article, we will concentrate on understanding the fundamental approaches behind this concept without delving into the detailed technical specifics of its implementation. The computing and storage resources for the sub0layer are planned to be sourced from community devices, augmented by a core infrastructure of root servers, which we refer to as "Nano Data Centers". Initially, this foundational infrastructure will be established by LogosLabs. This setup allows the sub0layer to leverage a distributed network of resources, reducing reliance on centralized facilities and enhancing the robustness and resilience of the Web3 infrastructure. By integrating resources from a wide range of devices across the network, the sub0layer aims to distribute data processing and storage tasks, which aligns with the decentralized ethos of the Web3 movement. This architecture is designed to ensure that the Web3 ecosystem can operate more autonomously, minimizing potential control or interference from centralized entities while enhancing user privacy and data security.
This approach speaks to a critical issue within the Web3 ecosystem - the shift from relying on large cloud providers to harnessing computing resources provided by the community. Setting up and configuring a validator within the blockchain infrastructure requires a high level of expertise and several dependencies, making it less accessible compared to simply utilizing the computing power of personal computers, laptops, or private servers on a temporary basis. Utilizing community hardware through the sub0layer not only allows more individuals to participate in the provision of Web3 infrastructure but also helps avoid the dependency that comes with moving of the blockchain infrastructure to traditional cloud services. The question then arises whether such powerful hardware, typically found in data centers and often associated with high costs for resources that may be underutilized, is truly necessary for Web3 applications. Many private devices today are equipped with capable and efficient hardware. Particularly, gaming PCs, which often feature not just powerful GPUs but also high-performance CPUs and fast memory, can be utilized. The real challenge lies in leveraging these devices in a way that is technically efficient and secure. This is a key issue that needs to be addressed to ensure that community-provided resources can be safely and effectively integrated into the Web3 infrastructure.
Another key principle of the network is its "autonomy"; it is designed to function without human intervention by automatically maintaining and operating its infrastructure according to predefined rules. Decisions on future changes to the network will be governed by a community governance system that is grounded in the principles of fairness. The main implementation and regulation of the entire network will take place on a public blockchain, integrating a security model that allows private elements to operate securely in a public environment without private parties. This approach encourages broader and fairer participation and suggests that the sub0layer could play a significant role in the evolution of Web3.
The introduction of a decentralized, community-managed sub0layer (computational layer) in blockchain technology could address several challenges currently faced in the areas of validation and transaction fees. By setting a standardized, community-defined base price for computing power, the sub0layer could offer greater predictability and stability in computing costs. Democratizing access to computing resources on Web3 and offering them at standardized prices could significantly enhance decentralization, supporting smaller actors by enabling them to stay "competitive". This aligns with the fundamental principles of blockchain technology, which are centered around the distribution and decentralization of "power". As the price for computing power would be controlled by the community, it could be flexibly adjusted in response to changes in demand or the costs of providing services. This approach could foster a more balanced and equitable model that caters to the needs of both users and providers of computing power. A stabilized and community-controlled base price for computing power could also help to mitigate the volatility of transaction fees, which are often subject to speculative or sudden market fluctuations. This stabilization could enhance predictability for businesses and developers looking to utilize blockchain platforms, providing a more reliable approach for planning and investing in blockchain infrastructure.
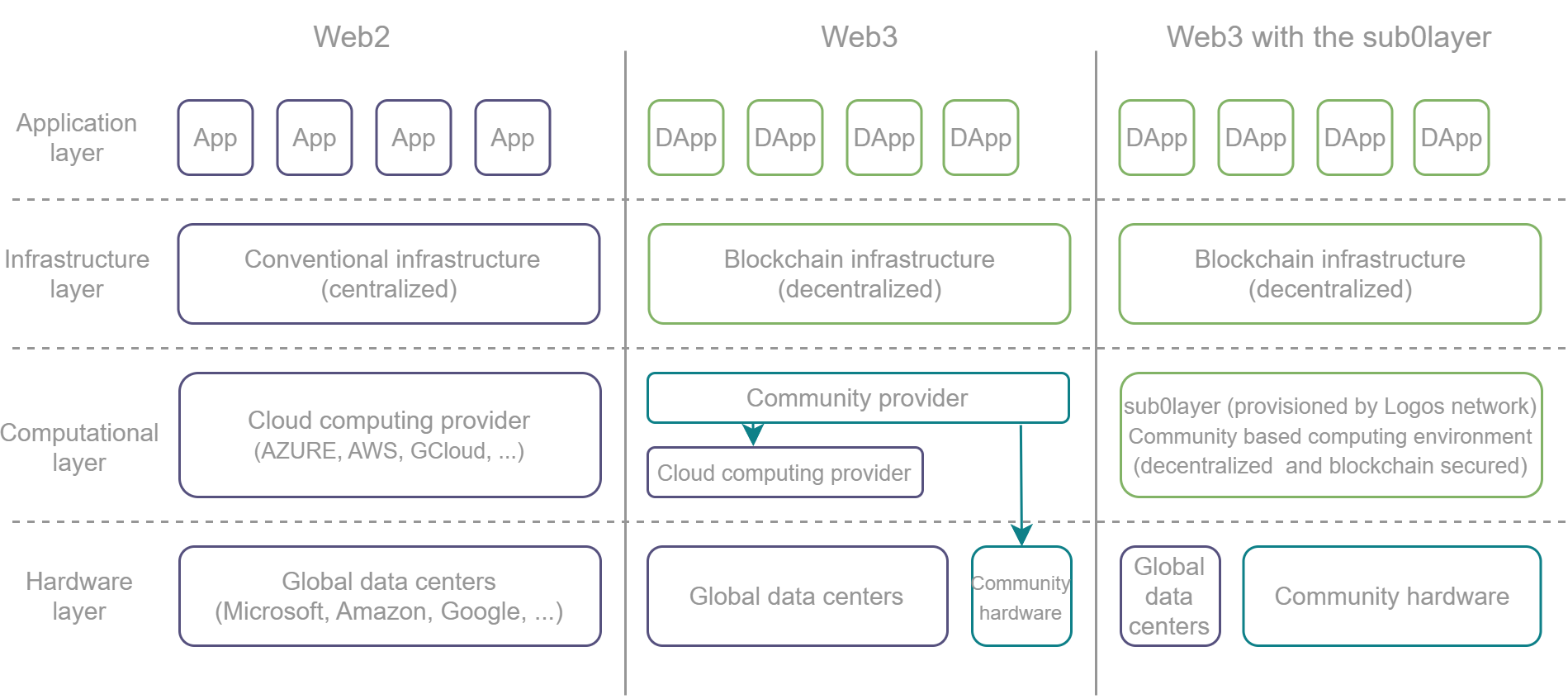
Fundamental overview of a Web2 approach in comparison to Web3, a Web3 with a new security and compute layer.
The sub0layer is envisioned as a computational layer within the Web3 ecosystem, designed to support the operation of blockchain nodes independent of their specific blockchain technologies, as it functions at the computational level. This implementation on the sub0layer allows for diverse approaches and solutions to challenges within the Web3 blockchain infrastructure. The operational environment for blockchain nodes on the sub0layer will be community-driven, utilizing blockchain technology to provide a more decentralized, transparent, and cost-efficient alternative to traditional cloud providers. Control over the "balance of power" will be maintained through a community governance system, enhancing the democratic ethos of the network. An important aspect of this approach is the expansion of the participant base by enabling the provision of pure computing power. This inclusivity allows even those not typically engaged with Web3 technologies to contribute technically to the Web3 ecosystem without the complexities of setting up a validator, as every resource provided supports the Web3 ecosystem (if not with validation, then with computation). A central tenet of the sub0layer is its adherence to zero trust and zero knowledge principles, which are crucial for creating a secure operating environment. The technical realization of these principles involves various methods, including the use of zero-knowledge proofs (SNARKs/STARKs/Binius), the implementation of access control methods (RBAC/ABAC/PBAC), the use of technical accounts and secure private key management. The philosophy of "no human interaction necessary" underpins the creation of an automated solution where security and operation are minimally affected by human factors, thereby reducing the risk of errors. This approach aims to leverage automation to ensure that the infrastructure is both robust and capable of self-management, further reducing potential vulnerabilities and inefficiencies associated with human intervention.
The conception and definition of the sub0layer represent a significant endeavor that demands meticulous planning and detailed specifications. As I mentioned in the conclusion of the previous article (opens in a new tab), we are faced with a significant amount of work. Our primary focus currently is on developing a robust security model, which is a crucial prerequisite for implementing a project as ambitious as the sub0layer. This model will ensure that as we build out the computational layer, it remains secure from vulnerabilities and capable of supporting the decentralized and autonomous functions vital for the Web3 ecosystem. The successful integration of such a security model will pave the way for further developments and enable us to move closer to realizing the full potential of the sub0layer.
Do you have questions or want to discuss more? Join our discussion on Discord (opens in a new tab)
References:
- Astar Docs - https://docs.astar.network (opens in a new tab)
- An interconnected network of sovereign blockchains - https://cosmos.network/whitepaper (opens in a new tab)
- CoreWeave's $1.1B raise shows the market for alternative clouds is booming - https://techcrunch.com/2024/05/05/coreweaves-1-1b-raise-shows-the-market-for-alternative-clouds-is-booming (opens in a new tab)
- Danksharding - https://ethereum.org/en/roadmap/danksharding (opens in a new tab)
- EIP-4844: Shard Blob Transactions - https://eips.ethereum.org/EIPS/eip-4844 (opens in a new tab)
- Multi-million dollar Cheyenne supercomputer auction ends with $480,085 bid - https://www.tomshardware.com/tech-industry/supercomputers/multi-million-dollar-cheyenne-supercomputer-auction-ends-with-480085-bid (opens in a new tab)
- Polkadot: Vision for a heterogeneous multi-chain framework - https://assets.polkadot.network/Polkadot-whitepaper.pdf (opens in a new tab)
- Polygon Whitepaper 1.0 https://whitepaper.io/document/646/polygon-whitepaper (opens in a new tab)
- Polygon Whitepaper 2.0 https://polygon.technology/papers/pol-whitepaper (opens in a new tab)
- The Internet Computer for Geeks - https://internetcomputer.org/whitepaper.pdf (opens in a new tab)
- The Big Cloud Exit FAQ - https://world.hey.com/dhh/the-big-cloud-exit-faq-20274010 (opens in a new tab)
- What Is Layer 0 in Blockchain? - https://academy.binance.com/en/articles/what-is-layer-0-in-blockchain (opens in a new tab)
- What is Blockchain Layer 0, 1, 2, 3 Explained - https://medium.com/@learnwithwhiteboard_digest/what-is-blockchain-layer-0-1-2-3-explained-56226d4bb2cd (opens in a new tab)
- What is the Blockchain Trilemma? - https://www.ledger.com/academy/what-is-the-blockchain-trilemma (opens in a new tab)